Thank you for visiting nature.com. You are using a browser version with limited support for CSS. To obtain the best experience, we recommend you use a more up to date browser (or turn off compatibility mode in Internet Explorer). In the meantime, to ensure continued support, we are displaying the site without styles and JavaScript.
Scientific Reports volume 12, Article number: 14656 (2022 ) Cite this article Erbium Oxide Powder

In recent times, biopolymer-metal oxide nanocomposites have gained prominent importance in the attenuation of environmental toxicants from aqueous phase. But lanthanide oxide-based biopolymer nanocomposites have scantly been evaluated for their adsorption potential. A novel guar gum-polyacrylamide/erbium oxide nanocomposite (GG-PAAm/Er2O3 NC) adsorbent was synthesized by copolymerization of guar gum (GG) and acrylamide (AAm) utilizing N-N′-methylenebisacrylamide as a crosslinker and Er2O3 as a reinforcing agent. The adsorptive efficacy of GG-PAAm/Er2O3 nanocomposite was evaluated using nile blue (NB) as a model pollutant dye from aquatic system. The prepared adsorbent was characterized by Fourier transform infrared (FTIR) spectroscopy, X-ray diffraction (XRD) analysis, Brunauer–Emmett–Teller (BET) analysis, thermogravimetric analysis, scanning electron microscopy-energy dispersive X-ray spectroscopy (SEM–EDX), and high-resolution transmission electron microscopy (HRTEM). The optimal process parameters, which include dosage (0.8 g/L), agitation time (40 min), initial solution pH (6), and initial NB concentration (80 mg/L) were determined by batch methodology. The equilibrium data for NB confiscation was better expressed by Langmuir isotherm model, with maximal adsorption effectiveness (Qm) of 225.88 mg NB/g demonstrating the actively monolayer adsorption onto homogeneous surface of GG-PAAm/Er2O3 NC. The kinetics of NB sorption process onto GG-PAAm/Er2O3 NC was reliable with pseudo-second order model. Thermodynamic parameters such as ΔH° (15–17 kJ/mol) and ΔS° (0.079–0.087 kJ/mol/K), and − ΔG° (8.81–10.55 kJ/mol) for NB validated the endothermic, an increased randomness at the GG-PAAm/Er2O3–NB interface, and spontaneity and feasibility of the process, respectively. The spent nanocomposite was effectively regenerated with NaOH, and could be reused proficiently for five runs demonstrating the high reusability potential of the nanocomposite. The commendable removal efficiency and high reusability of GG-PAAm/Er2O3 NC recommended it to be a highly competent adsorbent for cationic dyes particularly NB diminution from aqueous waste.
The control of environmental deterioration of aquatic resources resulting from the amplified discharge of liquid effluents due to rapid industrial development and global population growth has emerged as one of the major challenging tasks over the past several decades. Many industries such as textile, leather, tannery, cosmetics, paints or plastics are prime contributors of colored pollutants. The vast usage of synthetic dyes, which are mostly poorly-degradable and environmentally persistent due their complex molecular structures, in the textile industries emancipates huge quantity of dyes-laden water post dyeing and finishing operations. The release of unspent dyes in the receiving bodies not only worsens the water quality but presents a detrimental outcome including impaired photosynthesis of aquatic ecosystems, and carcinogenic, mutagenic or teratogenic influence on aquatic biota and humans1,2,3. Most dyes, on ingestion, are responsible for several diseases such as dizziness, vomiting, tremors, nausea, cyanosis and jaundice. Direct contact may result into allergic problems, skin irritation, and eye burn that can permanently damage the cornea. If inhaled, they may lead to breathing difficulty, profuse sweating, abdominal pain and hyper motility2. In the last few decades, much attention has been focused on mitigating the colored contaminants with a view to protecting the environmental sustainability and reduce the grave health risks associated with the industrial dyes4,5. Nile blue (NB) is an azo dye widely applied for dyeing in the textile industries. Many health-related problems including skin irritation, dermatitis, allergic reactions in eyes and respiratory disease are associated with its presence in water6. It can cause sleepiness, digestive system stimulation, cold feelings, mouth and throat irritation, redness and dryness of the skin, and chromosomal aberrations. Therefore, it is essential to decolorize the effluents containing the hazardous nile blue effectively before discharging into the aquatic system7.
The most common approaches for confiscating and decolorizing dyes from aquatic system are chemical coagulation, ozonolysis, photocatalysis, microbial degradation, electrochemical methods, and adsorption8,9,10. But most physico-chemical techniques are rather expensive, operationally cumbersome and energy-intensive. However, adsorption technology has attained widespread recognition as the most encouraging, reliable, highly effective, operationally simpler, efficient and cheap practice for environmental pollutants abatement with admirable regenerative and recyclable potential of the adsorbents.
Multitude of adsorbents such as activated carbons10, zeolites11, graphene oxides12, and clays13 have arduously been used for dyes decontamination. However, these adsorbents lack the traits of an ideal adsorbent, which has led to the pursuit for advanced adsorbents possessing higher sorption efficiencies. Over the recent years, biopolymers as adsorbents have gained popularity due to their environment-friendly characteristics14. However, due to their poor mechanical strength and fair water solubility, their usage in water pollution remediation is rather restricted. The utilization of metal oxides as filler in polymer matrices have minimized the strength related problem and has broadened their scope in water treatment. Metal oxides-based gum polysaccharide nanocomposites incorporating the synergistic assets of both the components have aroused a great deal of interest in aquatic environmental contaminants sequestration15,16,17 owing to their cheap and facile obtainability, feeble-toxicity, environmentally benign nature, improved surface area to volume ratio, easy modifiability, better biocompatibility, excellent mechanical strength, and magnificent adsorption effectiveness17,18,19,20. Many nanocomposite adsorbents derived from gum arabic21, gum karaya22, gum ghatti23, gum xanthan24, gum tragacanth25 have recently been described in the literature for sequestration of organic dyes from aquatic environment.
The modification of biopolymers is usually undertaken to impart certain functional groups/moieties to improve upon the surface characteristics and adsorptive performance. The nanocomposites based on guar gum (GG), a low-priced, innocuous, and biodegradable biopolymer, have shown promising applications as effective adsorbents for a number of organic and inorganic aquatic contaminants in the recent times26,27,28,29,30. However, to our best understanding, the adsorption studies on biopolymer/lanthanide oxide (nano)composites for the attenuation of diverse contaminants have hitherto received scant consideration. Recent researches on the removal of salicylhydroxamic acid and acid blue 92 by Nd2O331,32, rhodamine B by r-GO/rare earth metal oxide aerogels33, reactive black 5 using LaFeO3/chitosan nanocomposite34, arsenite by carrageenan-embedded LaFeO3 nanocomposite35, and metal ions by ceria and its composites36 have been undertaken. Additionally, grafted/cross-linked GG composites, Ag NPs containing GG/poly(AA) grafted polymer37, guar gum grafted acrylic acid/nanoclay38, mGG-PAAm39, GG/magnetite/chitosan40, pectin-crosslinked-GG/SPION41, GG-crosslinked-graphene oxide hydrogel42 and GG-metal oxide (nano)composites, ZnO NPs/GG43, Fe3O4-GG44, and TiO2/GG hydrogel45 have been arduously employed for the confiscation of many environmental pollutants. However, adsorption studies invoking guar gum cross-linked with PAAm (polyacrylamide) using N, N-methylenebisacrylamide as a cross-linker and erbium oxide as the filler has not been attempted so far to the best of our knowledge. Further, its utilization as an adsorbent for nile blue is also undocumented.
The present investigation aims at developing a novel and recyclable nanocomposite adsorbent for organic dyes remediation based on erbium oxide-reinforced guar gum-polyacrylamide biopolymer matrix and to evaluate its adsorption competence towards an organic cationic dye. The synthesis of guar gum-polyacrylamide/erbium oxide nanocomposite (GG-PAAm/Er2O3 NC), its characterization through infrared and nuclear magnetic resonance spectroscopy, X-ray diffraction, N2 adsorption/desorption, and thermogravimetric analyses, and scanning and transmission electron microscopy, and its liquid phase removal efficacy for nile blue (NB) is depicted in the current study. The process variables (agitation time, initial solution pH, dosage, and initial NB concentration) impacting the NB removal was explored to ascertain the optimal operating conditions, and various facets of the adsorptive uptake phenomena was explicated in terms of isotherm and kinetic parameters deduced by applying non-linear regression analyses of the adsorption data using the corresponding model equations. Thermodynamics parameters were also examined to estimate the energetic changes accompanying the adsorption process. Several adsorption–desorption cycles were performed in order to estimate the reuse potential of the adsorbent.
Guar gum (Loba chemie, India), Acrylamide (Spectrochem, India; 99.5%), N,N′-methylenebisacrylamide (MBA; Loba chemie, India; 99%), nile blue (Himedia, India; 96%), ammonium persulfate (APS) (Merck, India; 98%) were used as acquired without further purification. All chemicals were of AR standard. Double distilled water was employed for the preparation of working solutions. The operating pH of test solutions was adjusted between 2 and 10 by applying dilute HCl and NaOH solution (0.1 M). The FTIR spectra, XRD pattern, SEM images and TEM micrographs were obtained using Perkin-Elmer spectrometer, model BX spectrum, USA, Philips Analytica PW 1830 apparatus, (Philips, The Netherlands), Carl Zeiss JOEL scanning electron microscope, (Sigma 5.05, Germany) and Transmission electron microscope, HRTEM 200 kV model, FEI (Tecnai), respectively. TGA analyses (35 oC–600 oC) were performed using a Perkin-Elmer thermal analyser (TGA 4000, Massachusetts, USA, Pyris 6 TGA with PyrisTM software V. 11.0.0.0449) at a heating rate of 10 oC min–1 under nitrogen atmosphere. The initial and residual NB concentration was determined using a UV-visible spectrophotometer (T-80 + UV/Vis spectrophotometer, PG Instrument Ltd, UK).
The GG-PAAm/Er2O3 nanocomposite was prepared by cross-linking guar gum and acrylamide by employing MBA as a cross-linker in the presence of Er2O3 (filler). Aqueous suspension (100 ml) of guar gum (1.0 g), prepared by vigorous stirring for 1 h, and acrylamide solution (0.3 g in 10 mL of deionized water) were homogenously mixed under constant stirring at 60 °C followed by the addition of APS (1.0 g in 5 mL water) as initiator and Er2O3 as filler. N,N′-Methylenebisacrylamide (0.2 g) was added to the mixture under continuous stirring for 30 min, and the reaction mixture was sonicated for 1 h. The obtained product (%yield = 72) was washed with water and ethanol to remove unreacted moieties, oven-dried at 90 °C for 48 h, ground to a fine powder, and stored in a stoppered glass vial for further studies.
The impact of different operating factors such as dosage, agitation time, initial solution pH, initial NB concentration, and temperature on removal efficiency of GG-PAAm/Er2O3 nanocomposite adsorbent was evaluated by batch adsorption experiments, wherein NB solution (50 mL) of known concentration (20, 30, 40, 50 mg/L) was agitated in a water bath shaker with desired adsorbent mass (0.2–1.2 g/L) for different contact time (10–60 min) at constant temperature. After agitating for the desired time interval, the solution was centrifuged and the [NB] in the supernatant was determined spectrophotometrically at maximum absorbance wavelength of 635 nm. Adsorption isotherm studies at different temperatures (303, 308, and 313 K) was accomplished by shaking NB solution of various concentrations (30–80 mg/L) till equilibrium was achieved. However, for kinetics appraisal, similar experiments were conducted with optimal nanocomposite dosage (0.8 g/L) at 303 K and pH 6 using varying [NB] (30, 40 and 50 mg/L) for optimal agitation time (40 min).
The FTIR spectra of GG-PAAm/Er2O3 nanocomposite, prior and post adsorption, is shown in Fig. 1. The diminished intensity of peaks around 3000–3500 cm−1 for GG-PAAm/Er2O3 NC signified the interaction of hydroxyl groups of guar gum with amide group of polyacrylamide46. The peaks detected in the spectra around 1200 cm−1 depicted the C–C–O, C–OH and C–O–C stretching modes of polysaccharides14. The peaks at 1654 cm−1 and 1604 cm−1 were due to C=O stretching vibrations in acrylamide47. The peak observed at 1081 cm−1 was attributed to bending vibration for CH2–O–CH248, whereas the band at 1410 cm−1 due to C–N stretching vibrations49. The peak at 3028 cm−1 was ascribed to NH2 stretching vibrations of the polyacrylamide50. Additionally, the band at 659 and 608 cm−1 were attributed to Er–O–Er and Er–O linkage, respectively51, which clearly indicated the existence of metal–oxygen bond participating in the biopolymer nanocomposite. After NB adsorption (Fig. 1b), the FTIR spectrum showed slight shift in the peak position assigned to NH2 from 3028 to 3181 cm−1, and diminution of intensities together with slight shifting of absorption bands at 1654 and 1081 cm−1 to lower wavelengths indicating that the relevant functional groups were involved in the adsorption procedure either by van der Waals forces or hydrogen bonding9. Further, a slight shift in the O–H, C–O, and C–N bands; and a drop in the intensity of O–H stretching vibration at 3200 cm−1 after NB sorption, confirmed the interaction of dye molecules with existing functional groups10. Appearance of a new peak at 2934 cm−1 because of CH2 group of the aromatic ring structure of dye validated the sorption process onto the nanocomposite52.
FTIR spectra of (a) GG-PAAm/Er2O3 nanocomposite, and (b) NB-loaded nanocomposite.
The XRD diffraction pattern of Er2O3 is depicted in Fig. 2a that illustrated high crystallinity and sample purity. The main reflections of Er2O3 centred at 2ϴ = 19.56 (211), 28.60 (222), 32.82 (440), 42.72 (134), 48.54 (440), and 56.98 (622) matched completely with the JCPDS file no. 78–0390 having space group of Ia-353. The existence of characteristic peaks for erbium oxide in the diffraction pattern along with some shifting of the diffraction peaks and the formation of a semi-crystalline network due to the amalgamation of Er2O3 into the amorphous guar gum phase signified the fabrication of GG-PAAm/Er2O3 NC (Fig. 2b). Average crystallite size of GG-PAAm/Er2O3 NC was 57 nm.
XRD spectra of (a) Er2O3, (b) GG-PAAm/Er2O3 nanocomposite, (c) N2 adsorption–desorption curve, and (d) pore size distribution.
The N2 adsorption/desorption isotherm was used to determine the specific surface area and porosity of the nanocomposite. Higher surface area is related to greater accessibility of adsorptive sites, hence boosted adsorption aptitude of an adsorbent. The N2 adsorption/desorption isotherms of GG-PAAm/Er2O3 NC is shown in Fig. 2c,d. The specific surface area, pore volume and pore diameter of nanocomposite were 70 m2/g, 0.024 cm3/g and 5.796 nm, respectively. The pore diameter in the range of 2–50 nm confirmed the mesoporous nature of the prepared NC.
The thermal stability and rate of decomposition of the nanocomposite was gauged by TGA. This analysis could manifest the change in mass of the sample throughout the heating process. The TGA curves of GG and GG-PAAm/Er2O3 NC are shown in Fig. S1 revealing that the decomposition occurred in two stages. The first decomposition stage of GG started at 68.6 °C and ended at 168.9 °C with a loss of 10.13%, while in GG-PAAm/Er2O3 NC it started at 86.3 °C and terminated at 199.8 °C with a loss of 2.1%. The logical explanation for these results could be the moisture content loss associated with the gum. The second phase corresponding to the decomposition of sugars in GG started at 255.9 °C and terminated at 340.7 °C with a weight loss of 42%, while in GG-PAAm/Er2O3 this phase began at 264.7 °C and lasted till 342.9 °C with a weight loss of 38%. The results portrayed that the nanocomposite with Er2O3 as filler has acquired higher thermal stability with lesser mass loss than the parent gum.
The SEM micrographs along with related EDX spectra of GG-PAAm/Er2O3 NC and NB-loaded GG-PAAm/Er2O3 NC are presented in Fig. 3a,b. The morphology of GG-PAAm/Er2O3 NC displayed an irregular, uneven rough surface with heterogenous porous structures probably because of the cross-linking network suitable for adsorption. The existence of sufficient pores of different sizes and shapes were primarily accountable for greater surface area and admirable adsorption efficacy of GG-PAAm/Er2O3 NC. Figure 3b of GG-PAAm/Er2O3 NC, after confiscation of NB, exhibited almost smooth texture confirming the adsorption of NB onto GG-PAAm/Er2O3 NC. The EDX spectra for corresponding SEM micrographs are also revealed in Fig. 3a,b. The existence of C, N in the EDX spectra of NB-sorbed GG-PAAm/Er2O3 NC recommended the successful confiscation of NB onto the adsorbent surface. Considerable changes in the surface morphology of GG-PAAm/Er2O3 NC occurred after the sequestration of NB. The pores nearly disappeared, which might possibly be due to the occupation and entrapment of NB molecules in the pore structures.
SEM micrographs of (a) GG-PAAm/Er2O3 nanocomposite, and (b) NB-loaded nanocomposite along with corresponding EDX spectra.
To examine the structural morphology of GG-PAAm/Er2O3 NC, TEM investigation was performed. Figure 4a displays the TEM image for GG-PAAm/Er2O3 NC. The average size of GG-PAAm/Er2O3 NC was determined employing Image J software. The particle size distribution curve (Fig. 4b) suggested that the typical particles size ranged from 60 to 70 nm, that was in good agreement with XRD data. Moreover, the TEM image also confirmed the efficacious incorporation of Er2O3 within the biopolymer matrix. The grey portions in Fig. 5a demonstrated the GG and PAAm matrix, while the darker portions were accredited to Er2O3 nanoparticles randomly distributed into the GG-PAAm polymer matrix. In addition, such morphological features offered the GG-PAAm/Er2O3 NC a larger surface area.
TEM micrographs of (a) GG-PAAm/Er2O3 nanocomposite, and (b) histogram.
Effect of operational parameters on the removal of NB: (a) dose, (b) contact time, (c) initial concentration, and (d) initial solution pH.
The sorption characteristics of the nanocomposite for NB elimination was investigated in terms of different influencing parameters such as adsorbent dosage, initial NB concentration, agitation time, and initial solution pH at 303 K.
The reliance of aqueous phase removal of NB on variation in dosage was explored in 0.2–1.2 g/L range at pH 6, and the data is presented in Fig. 5a. The %R initially increased from 93.87 to 97.37 with an increment in adsorbent loading up to 0.8 g/L due to greater available active sites onto nanocomposite surface or an overall improved surface area, followed by a slow decrease thereafter. This decline in uptake efficacy on further increment in dosage might be due to higher accessible active sites relative to the number of NB molecules54. The removal capacity, however, showed an opposing trend with increase in adsorbent loading, which might be attributed to the overlapping of active sites, and/or decrease in surface area due to aggregation of adsorbent particles at higher dose that limited the removal capacity54,55. Therefore, 0.8 g/L of nanocomposite was selected as optimal dose for further studies. A similar trend of improved confiscation efficacy for NB with an increment in dosage of AC/CoFe2O4 nanocomposite56 and CuWO4 nanoparticles57 are described in the literature.
The agitation time is critical and significant factor in removing perilous pollutants. The effect of shaking time (10–60 min) on the sorbed NB onto the nanocomposite (0.8 g/L) is depicted in Fig. 5b. A rapid adsorption of NB occurred in the first 30 min (94.87–96.95%) suggesting a fast confiscation rate of NB molecules, gradually attaining equilibrium at 40 min with 97.62% removal. So, 40 min was preferred as the optimal equilibrium time for further studies. Initially, the removal process was rapid because of the existence of sufficient active surface-sites for absorbing NB. Subsequently, a decrement in the adsorption rate on increasing the shaking time was associated with the saturation of surface-active sites leading to decrease in the adsorption effectiveness. Similar trend in the NB removal was reported for clay/starch/MnFe2O458 and CNT/MgO/CuFe2O4 magnetic composite59 but with relatively higher equilibrium time of 60 min and 50 min, respectively.
The impact of change in the initial NB concentration (30–80 mg/L) on removal process was examined using optimal GG-PAAm/Er2O3 NC dosage (0.8 g/L) and time (40 min) at pH 6. The obtained results, depicted in Fig. 5c, revealed that the sequestration of NB declined from 97.08 to 95.93% with increasing initial NB concentration from 30 to 80 mg/L, which could be understood in terms of two adversative effects. A fixed mass of GG-PAAm/Er2O3 NC (0.8 g/L) has a definite number of surface-active sites. At low concentrations, the surface binding sites overwhelm the feeble number of NB molecules, which resulted in higher removal efficacy. However, when the solution concentration was increased further, the NB molecules progressively occupied the vacant sites, which reduced the number of available active sites. At high concentrations, fewer dye molecules occupied the remaining surface sites, hence decrease in removal efficiency. The increment in qe (36.40–95.93 mg/g) at higher solution concentration might be as a consequence of higher interactions between dye molecules and GG-PAAm/Er2O3 NC, or higher concentration gradient and/or increased driving force surpassing the mass transfer60. The optimal concentration for NB was 80 mg/L. Similar outcomes for NB adsorption with change in initial NB concentration were noticed for iron oxide nanoparticles61 and acrylamide- or 2-hydroxyethyl methacrylate-based copolymeric hydrogels62.
The solution pH is a crucial factor that plays a significant role in the removal process of contaminants. The extent of sorption is controlled by surface charge of the nanocomposite and the ionization of sorbate species that are governed by the solution pH63. Thus, the impact of the solution pH on NB confiscation was scrutinized in 2–10 pH range at optimum operating conditions (Fig. 5d). The pH of the solution reflects the nature of the physicochemical interactions of the NB molecules and the active sites of the GG-PAAm/Er2O3 NC. The pKa value of NB is 9.27 revealing its existence in the cationic form in the studied pH range. The pHzpc (= 5.6) of the nanocomposite meant that the surface was positively charged at pH < pHzpc implying an electrostatic repulsion between positive GG-PAAm/Er2O3 NC surface and cationic NB, which might have declined the removal rate in the pH range of 2–6. However, as the %uptake was significantly affected, it implied that other type of interactions like hydrogen bonding and π–π interactions might be responsible for higher removal rate (82.43–90.6%) in the 2–6 pH range. Further increase in the pH changed the surface of nanocomposite to negative, which made electrostatic attractions to be a part of removal mechanism and the %uptake augmented to 95.66% till pH 9. After that, the dye became negative and electrostatic repulsion declined the %removal. Similar trend was depicted for NB adsorption onto MoO3/Ppy nanocomposite6.
The analyses of isothermal equilibrium data at constant temperature by applying different isotherm models provide efficacious perspective with regard to maximum sorption capacity, homogeneity or heterogeneity of the adsorbent surface, affinity of the adsorbent towards adsorbate, coverage type, energy of adsorption, and the mechanism of adsorption. The corresponding isotherm parameters were determined using Ce versus qe plots employing Langmuir (Eq. 1), Freundlich (Eq. 2), Temkin (Eq. 3) and D-R (Eq. 4) models.
Langmuir isotherm asserts the sorption of NB onto the nanocomposite surface with finite number of energetically equivalent surface-active sites64 having equal affinity for NB molecules leading to monolayer formation.
Here, Ce (mg/L) and qe (mg/g) is the residual equilibrium NB concentration in fluid phase and the amount of NB sorbed onto the solid phase, respectively, Qm (mg/g) signifies maximum adsorption efficiency of the nanocomposite required to form monolayer of sorbate on its surface, b (L/mol) is a Langmuir constant, Kf (mg/g)(L/mg)\(^{{1}/{\text{n}_{\text f}}}\) is an indicator of sorption efficiency, nf indicates heterogeneity of nanocomposite surface and mutual interaction between sorbed species, 1/nf signifies functional strength of sorption, Kt (L/g) is binding constant associated with maximal binding energy, βt (= RT/bt) is a constant related to heat of adsorption, and qD (mg/g) is D–R sorption effectiveness.
The dimensionless factor, RL = \(\frac{1}{1+b{C}_{e}}\) is used to evaluate the feasibility and favorability of adsorption procedure.
The corresponding isotherm model parameters along with correlation coefficients (R2) and standard error of estimate (SEE) at the studied temperatures were estimated from qe versus Ce curves for Langmuir (Fig. 6a), Freundlich (Fig. 6b), Temkin (Fig. 6c) and D-R (Fig. 6d) isotherms, and are tabulated in Table 1. An increment in the computed Qm values from 195.16 to 225.88 mg/g with an increase in operating solution temperature (303–313 K) (Table 1) indicated an improvement in the adsorption aptitude of the nanocomposite at higher temperature probably resulting due to enhanced physical attachment between active binding sites and NB molecules, which designated the removal process as endothermic. The b parameter (0.061–0.069 L/g) varied in the order: 303 K < 308 K < 313 K, which accounted for the best NB-nanocomposite binding at higher temperature. The RL parameter (0.375–0.193) lying between zero to unity validated the energetically favorable sorption, and contemplated a strong NB-nanocomposite interaction14 probably accounting for high percentage elimination of NB. The parameter, Qm is used to evaluate the sorption potential of a given adsorbent. A considerably higher adsorption efficacy (Qm) of 225.88 mg NB/g at 313 K relative to most described adsorbents in the literature for NB removal (Table 2) and various tree gum-based nanocomposites for other dyes confiscation (Table 3) validated the admirable sorption effectiveness of GG-PAAm/Er2O3 NC.
Isotherm plots: (a) Langmuir, (b) Freundlich, (c) Temkin, and (d) D-R for NB uptake.
Freundlich isotherm contemplates adsorption onto the surface of adsorbent with heterogeneous distribution of binding sites. It also illustrates the sorption as non-ideal and reversible phenomena where sorbent has non-uniform affinity leading to the multilayer sorption80. The magnitude of the parameter, nf is an indicator of the sorbent surface heterogeneity, and its value close to unity expresses a higher surface heterogeneity. The value of 1/nf is a measure of favorable, unfavorable or irreversible sorption process. The 1/nf value < 0.5 specifies the facile sorption, 1/nf > 1.0 denotes cooperative adsorption, while 1/nf > 2 depicts that NB is hardly sorbed54. The values 1/nf below 0.5 (0.445–0.475) together with relatively higher Kf (57.88–61.44 (mg/g)(L/mg)1/nf) (Table 1) supported the positive and favorable sorption of NB. The increasing trend in Kf with rise in temperature confirmed the endothermic trait of sorption.
Temkin isotherm model is employed to investigate the interaction between sorbate and sorbent81. It takes into account that heat of adsorption for pollutants diminishes linearly instead of logarithmically with an increase in coverage of the nanocomposite surface. The equilibrium binding constant, Kt (L/g) values (4.84–5.46) displayed an incremental change with rise in temperature (303–313 K) pointing out a relatively enhanced electrostatic interaction between nanocomposite surface-sites and NB molecules at high temperature. An increment in the bT values, which is related to the heat of sorption from 0.024 to 0.041 kJ/mol (Table 1) testified to slightly higher bonding probability of NB at elevated temperature (313 K). Further, the endothermic physisorption of NB was evidenced by the positive bT values below 8 kJ/mol, which is confirmed by the pertinent thermodynamic parameter (∆H°)82.
Dubinin–Radushkevich (D–R) isotherm model adopts a pore filling sorption mechanism with multilayer character involving van der Waals interaction and is usually used to recognize the mode of adsorption, that is, physical or chemical83. It also provides reasonable evidence about the adsorption mechanism with possible distribution of energy onto non-homogenous surface of adsorbent. The mean free energy of adsorption (E) was deduced using the equation, E = \(\frac{1}{(2\upbeta )\frac{1}{2}}\) from the value of β (mol2/kJ2), estimated from Eq. (4). If the mean free energy, E is 1–8 kJ/mol then physical interaction governs the adsorption mechanism, while that between 8 and 16 kJ/mol indicates ion-exchange phenomenon. However, E > 16 kJ/mol specifies chemical interaction. The values of E equal to 0.081–0.091 kJ/mol advocated physisorption. The calculated values of qD (mg/g) were 139.74, 143.85, and 149.77 at 303, 308 and 313 K, which are in agreement with the similar trend in Qm values obtained using the Langmuir isotherm plot.
The estimation of excellent fit model is described on the basis of lower SEE and R2 values close to unity. It was concluded from Table 1 that Langmuir model with R2 near to one (0.975–0.995) and lower SEE (0.628–0.258) as compared to the values recorded for other isotherm models provided the best correlation of the data. The conformity of the equilibrium data to the Langmuir model implicated that the removal procedure occurred onto homogeneous surface of nanocomposite with the formation of monolayer coverage of NB molecules.
The adsorption kinetic models provide significant information on the rate of contaminant adsorption. These models are utilized to illustrate the experimental data to conclude the mechanism for the adsorption of contaminants from aquatic system at adsorbent–adsorbate interface. To interpret the adsorption procedure, the kinetic data was examined by pseudo-first order84 and pseudo-second order85 models by employing Eqs. (5) and (6), respectively:
where k1 (1/min) and k2 (g/mg/min) are adsorption rate constants for pseudo-first order and pseudo-second order kinetic model, respectively. The qe and qt signify adsorption capability of NB at equilibrium and time t, respectively. The values of k1, k2 and qe were determined for different initial NB concentration from the slope and intercept of the plot of qt versus t (Fig. 7a,b), and are presented in Table 4 along with R2 and SEE. The pseudo-second order model approved the best depiction of sorption data based on higher R2 (0.925–0.978) and lower SEE values (0.077–0.136), which indicated that the removal of NB by nanocomposite was affected by the number of active binding sites instead of the initial NB concentration. The appropriateness of the pseudo-second order kinetic model in describing the experimental data recommended that the rate limiting step for NB confiscation by GG-PAAm/Er2O3 NC probably involved chemisorption mechanism. The decrease in the magnitude of k2 values with increment in initial NB concentration (0.069–0.059 g/mg/min) (Table 4) signified rapid adsorption at lower concentration, which could be ascribed to the lesser competition faced by NB molecules for surface active-sites suggestive of physisorption54.
Kinetic plots: (a) pseudo-first order, (b) pseudo-second order, (c) intraparticle diffusion, and (d) liquid film diffusion for NB removal.
Interpretation of kinetic data is crucial to conclude the adsorption procedure governing the rate controlling steps. Usually, the liquid film diffusion implicating external mass transfer of NB molecules from bulk solution to GG-PAAm/Er2O3 NC surface, intraparticle diffusion and interior pore diffusion are encompassed in the adsorptive scavenging of dyes. The Boyd liquid film and Weber-Morris intraparticle diffusion models86,87 are respectively expressed as Eqs. (8) and (7)
where ki (mg/g/min0.5) and kD (1/min) are rate constants for intraparticle and liquid film diffusion, respectively and Ci is the intercept expressing the boundary layer thickness.
The straight-line curves of either qt versus t0.5 (Fig. 7c) or –ln(1–F) versus t (Fig. 7d) (F = qe/qt) at 40 mg/L and 50 mg/L initial NB concentration with Ci = 0 delineate that the dynamics of the confiscation process of NB is controlled either by intraparticle or liquid film diffusion as the rate-limiting step. The intraparticle diffusion graphs, however, deviated from the linearity with high boundary layer contribution to the rate-controlling step (Ci = 45.65 and 58.48) indicating that it did not solely control the adsorption rate. Similarly, the liquid film diffusion plots also were not linear and did not pass through the origin, which precluded the liquid film diffusion as the only rate governing step. The values of ki (mg/g min0.5) and kD (1/min) were 0.306–0.302 and 0.047–0.066, respectively at the studied concentrations. It could, therefore, be inferred that the adsorption process of NB was controlled by both the diffusion mechanisms. However, based on R2 and SEE values for intraparticle diffusion (0.985–0.987; 0.016–0.020) and liquid film diffusion (0.989–0.991; 0.003–0.007), it could be concluded that the liquid film diffusion has a predominant role.
Arrhenius equation (lnk2 = lnA − Ea/RT) was employed to determine the activation energy (Ea) for the adsorption of NB onto GG-PAAm/Er2O3 NC surface, where k2 is the pseudo-second order rate constant. The slope of lnk2 versus 1/T plot, displayed in Fig. 8a, gave the precise value of Ea,, and is listed in Table 5. Activation energy provides an insight about the nature of adsorption i.e., physical, or chemical. The low values of Ea (5–50 kJ/mol) are indicative of physisorption, whereas the Ea between 60 and 800 kJ/mol recommend chemisorption. Low values of Ea usually imply a process controlled by diffusion and greater values signify the involvement of chemical reactions. Therefore, the determined value (Ea = 15.33) advocated physisorption, which is consistent with the values reported in literature, which are 13.2 kJ/mol for adsorption of methyl violet onto perlite88 and 19.25 kJ/mol for maxilon blue 5G on sepiolite89.
(a) Arrhenius plot, (b) van’t Hoff plot, (c) reusability potential of GG-PAAm/Er2O3 nanocomposite.
The enhancement in NB removal on increasing the temperature from 298 to 313 K represented an endothermic adsorption process. The thermodynamic parameters such as changes in free energy (ΔG°), entropy (∆S°), and enthalpy (∆H°) are used to determine the feasibility, spontaneity, and nature of the sorption procedure. The parameters, ΔG°, ∆H°, and ∆S° were evaluated by employing Eqs. (9) and (10):
where kc = qe/Ce, R = universal gas constant (8.314 J/mol K), and T = absolute temperature (K).
The slope and intercept of the linear curves of logkc versus 1/T (Fig. 8b) provided the ΔH° and ΔS° values, respectively (Table 6). It has been reported that ∆H° values of 2–10 kJ/mol designates physisorption mechanism involving van der Waals interactions, between 2 and 40 kJ/mol denotes hydrogen bonding, while that above 60 kJ/mol deduces chemisorption90. The positive ∆H° (15.35–17.87 kJ/mol) directed that the sorption process was endothermic and involved physisorption. Moreover, positive ∆S° (0.079–0.087 kJ/mol/K) (Table 6) suggested an elevated randomness indicating an escalated degree of freedom at solid–liquid interface. Similarly, a decrease in the negative values of ΔG◦ (− 8.41 to − 9.67 kJ/mol) with increment in temperature advocated that the adsorption was spontaneous and more favorable at higher temperatures. It could, therefore, be concluded that the mechanism of NB sorption onto GG-PAAm/Er2O3 NC was mainly governed by physisorption at the studied temperatures (298–313 K).
The intention of regeneration is not only to recover the removal efficacy of spent adsorbent, but also to recycle and reuse the valuable adsorbent for several series of sorption–desorption without loss of efficiency and stability, which might be helpful in sustainable management of the waste adsorbents, and would cut the overall treatment cost. Since operating solution pH had significant impact on NB confiscation by GG-PAAm/Er2O3 NC, it was essential to control pH during desorption. For regeneration investigation, 2.0 g/L of GG-PAAm/Er2O3 NC was agitated with NB solution (50 mg/L) for 1 h, then 0.1 mol/L NaOH was utilized as an eluent. Figure 8c demonstrates the extent of NB removal by GG-PAAm/Er2O3 NC up to fifth sorption–desorption cycles. After fifth cycle, the R% diminished from 96 to 79, which might be explicated to the large number of reversible surface-sites of GG-PAAm/Er2O3 NC. The regeneration results pointed out that the GG-PAAm/Er2O3 NC was recyclable and extremely effective in eliminating NB signifying the excellent potential relevance of the adsorbent at industrial scale.
Viscometry method was used to appraise the biodegradability progress of GG-PAAm and GG-PAAm/Er2O3 NC. The advances in biodegradation was examined by assessing the intrinsic viscosity after every five days. From Fig. 9, it was apparent that both GG-PAAm and GG-PAAm/Er2O3 NC were susceptible to biodegradation. The solution depicted degradation in 5–50 days as the solution disclosed a significant loss in the viscosity, which suggested the biodegradable nature of the biopolymers. However, GG-PAAm/Er2O3 NC portrayed lesser degradability relative to GG-PAAm matrix, which might be due to an increased mechanical strength owing to erbium oxide doping.
Biodegradation curves of GG-PAAm and GG-PAAm/ Er2O3 nanocomposite.
The functional groups on the surface of GG-PAAm/Er2O3 NC, and the initial pH of the dye solution play significant roles in the adsorption of NB. The adsorption of pollutants onto different adsorbents generally occurs through various interactions such as electrostatic, hydrogen bonding, dipole–dipole, van der Waals forces and π–π. To explicate the mechanism accountable for the confiscation of NB by GG-PAAm/Er2O3 NC, pH and FTIR studies were utilized. The percentage removal of NB with pH suggested electrostatic interaction as one of the mechanisms responsible for NB uptake. The slight change in the position of IR spectral peak from 3028 to 3181 cm−1 and change in intensity of the vibrational bands at 1654 and 1081 cm−1 specified that the relevant functional groups were involved in the adsorption procedure through hydrogen bonding. However, the change in the peak intensities of O–H, C–O, and C–N groups confirmed the interaction of NB with existing functional groups. It might, therefore, be inferred that electrostatic interaction, hydrogen bonding and π–π interactions were mainly involved in the adsorption of NB onto GG-PAAm/Er2O3 NC surface. The plausible mechanism of NB sequestration by the GG-PAAm/Er2O3 NC is schematically illustrated in Scheme 1.
Schematic representation of the plausible interactions of NB with GG-PAAm/Er2O3 nanocomposite.
A novel GG-PAAm/Er2O3 nanocomposite with admirable adsorption capacity (225.88 mg NB/g) was efficaciously fabricated by an efficient, inexpensive, environmentally benign, and easy-to-use ultrasonic-assisted polymerization process. Fourier transform infrared spectroscopy, X-ray diffraction, scanning electron microscopy, energy dispersive X-ray, transmission electron spectroscopy, thermogravimetric analysis, specific surface area (SBET) and pHzpc measurements were used to characterize the synthesized nanocomposite, and was successfully employed for adsorptive elimination of nile blue from liquid phase. The surface area, pore volume and pore diameter of GG-PAAm/Er2O3 NC were 70 m2/g, 0.024 cm3/g and 5.796 nm, respectively. The adsorption parameters such as dose (0.8 g/L), concentration (80 mg/L), time (40 min) and pH (6) were optimized. The equilibrium data best fitted to the Langmuir isotherm model signifying homogenous sorption of NB onto the surface of GG-PAAm/Er2O3 NC. The high Qm value (225.88 mg NB/g) at 313 K validated better sorption competence of the GG-PAAm/Er2O3 NC for NB confiscation. The rate of NB sorption onto the sorbent surface was governed by pseudo-second order kinetic model with intraparticle and liquid film diffusion controlling the overall rate. The positive ΔH° (15.35–17.86 kJ/mol) suggested endothermic physisorption, whereas ΔS° (0.079–0.087 kJ/mol/K) indicated an increased randomness at the sorbent-NB solution interface. The negative ΔG° (− 8.41 to − 9.67 kJ/mol) governed the spontaneity and feasibility of the process. The regenerated adsorbent demonstrated good performance up to fifth cycles without much loss in efficiency, which implied that GG-PAAm/Er2O3 NC could be employed as an efficacious and potent adsorbent for cationic dyes including NB sequestration from waste water.
The datasets used and/or analysed during the current study available from corresponding author on reasonable request.
Lellis, B., Fávaro-Polonio, C. Z., Pamphile, J. A. & Polonio, J. C. Effects of textile dyes on health and the environment and bioremediation potential of living organisms. Biotechnol. Res. Innov. 3, 275–290 (2019).
Dutta, S., Gupta, B., Srivastava, S. K. & Gupta, A. K. Recent advances on the removal of dyes from wastewater using various adsorbents: A critical review. Adv. Mater. 2, 4497–4531 (2021).
Khan, I. S., Ali, M. N., Hamid, R. & Ganie, S. A. Genotoxic effect of two commonly used food dyes metanil yellow and carmoisine using Allium cepa L. as indicator. Toxicol. Rep. 7, 370–375 (2020).
Article CAS PubMed PubMed Central Google Scholar
Hussain, D., Siddiqui, M. F., Shirazi, Z. & Khan, T. A. Evaluation of adsorptive and photocatalytic degradation properties of FeWO4/polypyrrole nanocomposite for rose bengal and alizarin red S from liquid phase: Modeling of adsorption isotherms and kinetics data. Environ. Prog. Sustain. Energy 20, e13822 (2022).
Subhan, H., Alam, S., Shah, L. A., Ali, M. W. & Farooq, M. Sodium alginate grafted poly (N-vinyl formamide-co-acrylic acid)-bentonite clay hybrid hydrogel for sorptive removal of methylene green from wastewater. Colloids Surf. A Physicochem. Eng. Asp. 611, 125853 (2021).
Siddiqui, M. F., Khan, E. A. & Khan, T. A. Synthesis of MoO3/polypyrrole nanocomposite and its adsorptive properties toward cadmium(II) and nile blue from aqueous solution: Equilibrium isotherm and kinetics modeling. Environ. Prog. Sustain. Energy 38, e13249 (2019).
Khan, T. A., Rahman, R. & Khan, E. A. Adsorption of malachite green and methyl orange onto waste tyre activated carbon using batch and fixed-bed techniques: Isotherm and kinetics modelling. Model. Earth Syst. Environ. 3, 38 (2017).
Kumar, A. et al. Bio-inspired and biomaterials-based hybrid photocatalysts for environmental detoxification: A review. Chem. Eng. J. 382, 122937 (2020).
Kausar, A. et al. Preparation and characterization of chitosan/clay composite for direct rose FRN dye removal from aqueous media: Comparison of linear and non-linear regression methods. J. Mater. Res. Technol. 8, 1161–1174 (2019).
Liu, Z., Khan, T. A., Islam, M. A. & Tabrez, U. A review on the treatment of dyes in printing and dyeing wastewater by plant biomass carbon. Bioresour. Technol. 354, 127168 (2022).
Article CAS PubMed Google Scholar
Abukhadra, M. R. & Mohamed, A. S. Adsorption removal of safranin dye contaminants from water using various types of natural zeolite. Silicon 11, 1635–1647 (2019).
Mao, B., Sidhureddy, B., Thiruppathi, A. R., Wood, P. C. & Chen, A. Efficient dye removal and separation based on graphene oxide nanomaterials. New J. Chem. 44, 4519–4528 (2020).
Momina, S. M. & Isamil, S. Regeneration performance of clay-based adsorbents for the removal of industrial dyes: A review. RSC Adv. 8, 24571–24587 (2018).
Article ADS CAS PubMed PubMed Central Google Scholar
Dassanayake, R., Acharya, S. & Abidi, N. Recent advances in biopolymer-based dye removal technologies. Molecules 26, 4697 (2021).
Article CAS PubMed PubMed Central Google Scholar
Etemadinia, T., Barikbin, B. & Allahresani, A. Removal of Congo red dye from aqueous solutions using ZnFe2O4/SiO2/Tragacanth gum magnetic nanocomposite as a novel adsorbent. Surf. Interfaces 14, 117–126 (2019).
Kulal, P., Krishnappa, P. B. & Badalamoole, V. Development of gum acacia based magnetic nanocomposite adsorbent for wastewater treatment. Polym. Bull. 20, 1–28 (2021).
Hassanzadeh-Afruzi, F., Maleki, A. & Zare, E. N. Efficient remediation of chlorpyrifos pesticide from contaminated water by superparamagnetic adsorbent based on Arabic gum-grafted-polyamidoxime. Int. J. Biol. Macromol. 203, 445–456 (2022).
Article CAS PubMed Google Scholar
Thakur, S. et al. Synthesis and characterisation of zinc oxide modified biorenewable polysaccharides based sustainable hydrogel nanocomposite for Hg2+ ion removal: Towards a circular bioeconomy. Bioresour. Technol. 20, 126708 (2022).
Dong, K., Xu, K., Wei, N., Fang, Y. & Qin, Z. Three-dimensional porous sodium alginate/gellan gum environmentally friendly aerogel: Preparation, characterization, adsorption, and kinetics studies. Chem. Eng. Res. Des. 20, 25 (2022).
Kulal, P. & Badalamoole, V. Evaluation of gum ghatti-g-poly (itaconic acid) magnetite nanocomposite as an adsorbent material for water purification. Int. J. Biol. Macromol. 193, 2232–2242 (2021).
Article CAS PubMed Google Scholar
Mittal, H., Morajkar, P. P., Al Alili, A. & Alhassan, S. M. In-situ synthesis of ZnO nanoparticles using gum arabic based hydrogels as a self-template for effective malachite green dye adsorption. J. Polym. Environ. 28, 1637–1653 (2020).
Mittal, H., Babu, R., Dabbawala, A. A., Stephen, S. & Alhassan, S. M. Zeolite-Y incorporated karaya gum hydrogel composites for highly effective removal of cationic dyes. Colloids Surf. A Physicochem. Eng. Asp. 586, 124161 (2020).
Banerjee, S., Debnath, A. & Singh, V. Gum ghatti-alginate hybrid bead derived titania spheres for deep removal of toxic dye remazol brilliant violet from aqueous solutions. Environ. Nanotechnol. Monit. Manage. 15, 100459 (2021).
Mittal, H., Al Alili, A., Morajkar, P. P. & Alhassan, S. M. Graphene oxide crosslinked hydrogel nanocomposites of xanthan gum for the adsorption of crystal violet dye. J. Mol. Liq. 323, 115034 (2021).
Sharma, B. et al. Titania modified gum tragacanth based hydrogel nanocomposite for water remediation. J. Environ. Chem. Eng. 9, 104608 (2021).
Saya, L. et al. Guar gum based nanocomposites: Role in water purification through efficient removal of dyes and metal ions. Carbohydr. Polym. 261, 117851 (2021).
Article CAS PubMed Google Scholar
Hussain, D., Khan, S. A. & Khan, T. A. Fabrication and characterization of mesoporous guar gum/NiWO4 nanocomposite for efficient adsorption of phloxine B and crystal violet from aqueous solution and evaluation of its antioxidant activity. Colloids Interface Sci. Commun. 44, 100488 (2021).
Sharma, G. et al. Guar gum and its composites as potential materials for diverse applications: A review. Carbohydr. Polym. 199, 534–545 (2018).
Article CAS PubMed Google Scholar
Yan, L., Chang, P. R., Zheng, P. & Ma, X. Characterization of magnetic guar gum-grafted carbon nanotubes and the adsorption of the dyes. Carbohydr. Polym. 87, 1919–1924 (2012).
Khan, T. A., Nazir, M., Ali, I. & Kumar, A. Removal of chromium(VI) from aqueous solution using guar gum–nano zinc oxide biocomposite adsorbent. Arab. J. Chem. 10, S2388–S2398 (2017).
Sime, M., Das, A., Galt, G., Hope, G. & Young, C. Studies on the nature of salicyl hydroxamate adsorption at the surface of neodymium oxide. J. Dispers. Sci. Technol. 40, 1488–1498 (2019).
Ahmadi, S. et al. Acid dye removal from aqueous solution by using neodymium(III) oxide nanoadsorbents. J. Nanomater. 10, 556 (2020).
Zhang, Y., Li, K. & Liao, J. Facile synthesis of reduced-graphene-oxide/rare-earth-metal-oxide aerogels as a highly efficient adsorbent for Rhodamine-B. Appl. Surf. Sci. 504, 144377 (2020).
Marhalim, M. A. A. et al. Enhanced performance of lanthanum orthoferrite/chitosan nanocomposites for adsorptive photocatalytic removal of Reactive Black 5. Korean J Chem. Eng. 38, 1648–1659 (2021).
Mudhulkar, R., Damarla, K. & Pappula, V. N. Preparation and characterization of carrageenan-embedded lanthanum iron oxide nanocomposite for efficient removal of arsenite ions from water. Anal. Methods 14, 449–459 (2022).
Article CAS PubMed Google Scholar
Olivera, S. et al. Cerium dioxide and composites for the removal of toxic metal ions. Environ. Chem. Lett. 16, 1233–1246 (2018).
Singh, J. & Dhaliwal, A. S. Effective removal of methylene blue dye using silver nanoparticles containing grafted polymer of guar gum/acrylic acid as novel adsorbent. J Polym. Environ. 29, 71–88 (2021).
Shruthi, S. B., Bhat, C., Bhaskar, S. P., Preethi, G. & Sailaja, R. R. N. Microwave assisted synthesis of guar gum grafted acrylic acid/nanoclay superabsorbent composites and its use in crystal violet dye absorption. Green Sustain. Chem. 6, 11 (2016).
Hiremath, J. N. & Vishalakshi, B. Evaluation of a pH-responsive guar gum-based hydrogel as adsorbent for cationic dyes: Kinetic and modelling study. Polym. Bull. 72, 3063–3081 (2015).
Hamza, M. F. et al. Phosphorylation of guar gum/magnetite/chitosan nanocomposites for uranium(VI) sorption and antibacterial applications. Molecules 26, 1920 (2021).
Article CAS PubMed PubMed Central Google Scholar
Sharma, G. et al. Pectin-crosslinked-guar gum/SPION nanocomposite hydrogel for adsorption of m-cresol and o-chlorophenol. Sustain. Chem. Pharm. 6, 96–106 (2017).
Gopi, S., Rajeswari, A., Sudharsan, G. & Pius, A. Highly crosslinked 3-D hydrogels based on graphene oxide for enhanced remediation of multi contaminant wastewater. J. Water Process. Eng. 31, 100850 (2019).
Rezk, M. Y., Zeitoun, M., El-Shazly, A. N., Omar, M. M. & Allam, N. K. Robust photoactive nanoadsorbents with antibacterial activity for the removal of dyes. J. Hazard. Mater. 378, 120679 (2019).
Article CAS PubMed Google Scholar
Sahoo, J. K. et al. Guar gum-coated iron oxide nanocomposite as an efficient adsorbent for congo red dye. Desalin. Water Treat. 20, 342–354 (2017).
Santoso, S. P. et al. TiO2/guar gum hydrogel composite for adsorption and photodegradation of methylene blue. Int. J. Biol. Macromol. 193, 721–733 (2021).
Article CAS PubMed Google Scholar
Vanaamudan, A., Sadhu, M. & Pamidimukkala, P. Chitosan-Guar gum blend silver nanoparticle bio nanocomposite with potential for catalytic degradation of dyes and catalytic reduction of nitrophenol. J. Mol. Liq. 271, 202–208 (2018).
Sharma, G. et al. Gum acacia-cl-poly (acrylamide)@carbon nitride nanocomposite hydrogel for adsorption of ciprofloxacin and its sustained release in artificial ocular solution. Macromol. Mater. Eng. 305, 2000274 (2020).
Sharma, G. et al. Fabrication and characterization of novel Fe0@Guar gum-crosslinked-soya lecithin nanocomposite hydrogel for photocatalytic degradation of methyl violet dye. Sep. Purif. Technol. 211, 895–908 (2019).
Pal, S. et al. Modified guar gum/SiO2: Development and application of a novel hybrid nanocomposite as a flocculant for the treatment of wastewater. Environ. Sci. Water Res. Technol. 1, 84–95 (2015).
Khan, S. A., Siddiqui, M. F. & Khan, T. A. Ultrasonic-assisted synthesis of polyacrylamide/bentonite hydrogel nanocomposite for the sequestration of lead and cadmium from aqueous phase: Equilibrium, kinetics and thermodynamic studies. Ultrason. Sonochem. 60, 104761 (2020).
Article CAS PubMed Google Scholar
Azad, F. & Maqsood, A. Fabrication, structural characterization, dielectric and electrical parameters of the synthesized nano-crystalline erbium oxide. Electron. Mater. Lett. 10, 557–563 (2014).
Article ADS CAS Google Scholar
Boushehrian, M. M., Esmaeili, H. & Foroutan, R. Ultrasonic assisted synthesis of Kaolin/CuFe2O4 nanocomposite for removing cationic dyes from aqueous media. J. Environ. Chem. Eng. 8, 103869 (2020).
Rahimi-Nasrabadi, M. et al. Statistical optimization of experimental parameters for synthesis of two efficient photocatalyst: Erbium carbonate and erbium oxide nanoparticles. J. Mater. Sci. Mater. Electron. 28, 15224–15232 (2017).
Khan, T. A., Nouman, M., Dua, D., Khan, S. A. & Alharthi, S. S. Adsorptive scavenging of cationic dyes from aquatic phase by H3PO4 activated indian jujube (Ziziphus mauritiana) seeds based activated carbon: Isotherm, kinetics, and thermodynamic study. J. Saudi Chem. Soc. 20, 101417 (2022).
Arshadi, M., SalimiVahid, F., Salvacion, J. W. L. & Soleymanzadeh, M. Adsorption studies of methyl orange on an immobilized Mn-nanoparticle: Kinetic and thermodynamic. RSC Adv. 4, 16005–16017 (2014).
Article ADS CAS Google Scholar
Foroutan, R., Mohammadi, R. & Ramavandi, B. Elimination performance of methylene blue, methyl violet, and nile blue from aqueous media using AC/CoFe2O4 as a recyclable magnetic composite. Environ. Sci. Pollut. Res. 26, 19523–19539 (2019).
Kavitha, B. & Karthiga, R. Synthesis and characterization of CuWO4 as nano- adsorbent for removal of nile blue and its antimicrobial studies. J. Mater. Environ. Sci. 11, 57–68 (2020).
Esvandi, Z., Foroutan, R., Peighambardoust, S. J., Akbari, A. & Ramavandi, B. Uptake of anionic and cationic dyes from water using natural clay and clay/starch/MnFe2O4 magnetic nanocomposite. Surf. Interfaces 21, 100754 (2020).
Foroutan, R., Peighambardoust, S. J., Esvandi, Z., Khatooni, H. & Ramavandi, B. Evaluation of two cationic dyes removal from aqueous environments using CNT/MgO/CuFe2O4 magnetic composite powder: A comparative study. J. Environ. Chem. Eng. 9, 104752 (2021).
Zubir, M. H. M. & Zaini, M. A. A. Twigs-derived activated carbons via H3PO4/ZnCl2 composite activation for methylene blue and congo red dyes removal. Sci. Rep. 10, 1–17 (2020).
Kushwaha, R., Garg, S., Bajpai, S. & Giri, A. S. Degradation of Nile blue sulphate dye onto iron oxide nanoparticles: Kinetic study, identification of reaction intermediates, and proposed mechanistic pathways. Asia-Pac. J. Chem. Eng. 13, e2200 (2018).
Işikver, Y. Removal of some cationic dyes from aqueous solution by acrylamide- or 2-hydroxyethyl methacrylate-based copolymeric hydrogels. Fibers Polym. 18, 2070–2078 (2017).
Nayak, A. K. & Pal, A. Statistical modeling and performance evaluation of biosorptive removal of Nile blue A by lignocellulosic agricultural waste under the application of high-strength dye concentrations. J. Environ. Chem. Eng. 8, 103677 (2020).
Langmuir, I. The adsorption of gases on plane surfaces of glass, mica and platinum. J. Am. Chem. Soc. 40, 1361–1403 (1918).
İyim, T. B., Acar, I. & Özgümüş, S. Removal of basic dyes from aqueous solutions with sulfonated phenol–formaldehyde resin. J. Appl. Polym. Sci. 109, 2774–2780 (2008).
Maderova, Z., Baldikova, E., Pospiskova, K., Safarik, I. & Safarikova, M. Removal of dyes by adsorption on magnetically modified activated sludge. Int. J. Environ. Sci. Technol. 13, 1653–1664 (2016).
Safarik, I. et al. Magnetically-modified natural biogenic iron oxides for organic xenobiotics removal. Int. J. Environ. Sci. Technol. 12, 673–682 (2015).
Alipour, N. & Namazi, H. Removing Paraquat and Nile blue from aqueous solution using double-oxidized graphene oxide coated by polydopamine nanocomposite. Int. J. Environ. Sci. Technol. 16, 3203–3210 (2019).
Safarik, I., Horska, K. & Safarikova, M. Magnetically modified spent grain for dye removal. J. Cereal Sci. 53, 78–80 (2011).
Ghoochian, M. Adsorption of Nile blue A from wastewater using magnetic multi-walled carbon nanotubes: Kinetics and equilibrium studies. Iran. J. Toxicol. 10, 7–12 (2016).
Pandey, S., Do, J. Y., Kim, J. & Kang, M. Fast and highly efficient removal of dye from aqueous solution using natural locust bean gum based hydrogels as adsorbent. Int. J. Biol. Macromol. 143, 60–75 (2020).
Article CAS PubMed Google Scholar
Preetha, B. K. & Vishalakshi, B. Microwave assisted synthesis of karaya gum based montmorillonite nanocomposite: Characterisation, swelling and dye adsorption studies. Int. J. Biol. Macromol. 154, 739–750 (2020).
Article CAS PubMed Google Scholar
Chaudhary, S., Sharma, J., Kaith, B. S., Sharma, A. K. & Goel, A. Gum xanthan-psyllium-cl-poly (acrylic acid-co-itaconic acid) based adsorbent for effective removal of cationic and anionic dyes: Adsorption isotherms, kinetics and thermodynamic studies. Ecotoxicol. Environ. Saf. 149, 150–158 (2018).
Article CAS PubMed Google Scholar
Arun Krishna, K. & Vishalakshi, B. Gellan gum-based novel composite hydrogel: Evaluation as adsorbent for cationic dyes. J. Appl. Polym. Sci. 134(47), 45527 (2017).
Fosso-Kankeu, E., Mittal, H., Mishra, S. B. & Mishra, A. K. Gum ghatti and acrylic acid based biodegradable hydrogels for the effective adsorption of cationic dyes. Ind. Eng. Chem. Res. 22, 171–178 (2015).
Ghorai, S., Sarkar, A. K., Panda, A. B. & Pal, S. Effective removal of Congo red dye from aqueous solution using modified xanthan gum/silica hybrid nanocomposite as adsorbent. Bioresour. Technol. 144, 485–491 (2013).
Article CAS PubMed Google Scholar
Kaith, B. S., Jindal, R. & Sharma, R. Synthesis of a gum rosin alcohol-poly (acrylamide) based adsorbent and its application in removal of malachite green dye from waste water. RSC Adv. 5(54), 43092–43104 (2015).
Article ADS CAS Google Scholar
Sharma, G. et al. Fabrication and characterization of Gum arabic-cl-poly (acrylamide) nanohydrogel for effective adsorption of crystal violet dye. Carbohydr. Polym. 202, 444–453 (2018).
Article CAS PubMed Google Scholar
Bouaziz, F. et al. Efficiency of almond gum as a low-cost adsorbent for methylene blue dye removal from aqueous solutions. Ind. Crops Prod. 74, 903–911 (2015).
Freundlich, H. About adsorption in solutions, journal for physical chemistry.Z. Phys.Chem. 62, 121–125 (1906).
Temkin, M. I. Kinetics of ammonia synthesis on promoted iron catalysts. Acta Physiochim. URSS 12, 327–356 (1940).
Siddiqui, M. F. et al. A sugarcane bagasse carbon-based composite material to decolor and reduce bacterial loads in waste water from textile industry. Ind. Crops Prod. 176, 114301 (2022).
Dubinin, M. M. The equation of the characteristic curve of activated charcoal. In Dokl. Akad. Nauk. SSSR. 55, 327–329 (1947).
Corbett, J. F. Pseudo first-order kinetics. J. Chem. Educ. 49, 663 (1972).
Ho, Y. S. & McKay, G. Two-stage batch sorption and optimized design for dye removal. Chem. Eng. Trans. 76(Part B), 183–191 (1998).
Boyd, G. E., Adamson, A. W. & Myers, L. S. The exchange adsorption of ions from aqueous solutions by organic zeolites. II. Kinetics. J. Am. Chem. Soc. 69, 2836–2848 (1947).
Article CAS PubMed Google Scholar
Weber, W. J. Jr. & Morris, J. C. Kinetics of adsorption on carbon from solution. J. Sanit. Eng. Div. 89, 31–59 (1963).
Doğan, M. & Alkan, M. Adsorption kinetics of methyl violet onto perlite. Chemosphere 50, 517–528 (2003).
Article ADS PubMed Google Scholar
Alkan, M., Doğan, M., Turhan, Y., Demirbaş, Ö. & Turan, P. Adsorption kinetics and mechanism of maxilon blue 5G dye on sepiolite from aqueous solutions. Chem. Eng. J. 139, 213–223 (2008).
Abbasi, N., Khan, S. A., Khan, T. A. & Alharthi, S. S. Statistical evaluation of liquid phase sequestration of acridine orange and Cr6+ by novel mesoporous glutamic acid-g-polyacrylamide/plaster of paris/riboflavin hydrogel nanocomposite. Environ. Res. 213, 113712 (2022).
Article CAS PubMed Google Scholar
The author (DH) thanks the Ministry of Tribal Affairs, Govt. of India for award of Senior Research Fellowship, while (SSA) acknowledges the financial support through Taif University Researchers Supporting Project number (TURSP-2020/90), Taif University, Taif, Saudi Arabia.
Department of Chemistry, Jamia Millia Islamia, Jamia Nagar, New Delhi, 110 025, India
Daud Hussain, Suhail Ayoub Khan & Tabrez Alam Khan
Department of Chemistry, College of Science, Taif University, P.O. Box 110999, Taif, 21944, Saudi Arabia
You can also search for this author in PubMed Google Scholar
You can also search for this author in PubMed Google Scholar
You can also search for this author in PubMed Google Scholar
You can also search for this author in PubMed Google Scholar
D.H.: conceptualization, investigation, methodology, data curation, formal analysis, writing—original draft, S.A.K.: investigation, methodology, data curation, software, formal analysis, editing; T.A.K.: conceptualization, validation, editing, resources, supervision; S.S.A.: validation, editing, resources.
Correspondence to Tabrez Alam Khan.
The authors declare no competing interests.
Springer Nature remains neutral with regard to jurisdictional claims in published maps and institutional affiliations.
Open Access This article is licensed under a Creative Commons Attribution 4.0 International License, which permits use, sharing, adaptation, distribution and reproduction in any medium or format, as long as you give appropriate credit to the original author(s) and the source, provide a link to the Creative Commons licence, and indicate if changes were made. The images or other third party material in this article are included in the article's Creative Commons licence, unless indicated otherwise in a credit line to the material. If material is not included in the article's Creative Commons licence and your intended use is not permitted by statutory regulation or exceeds the permitted use, you will need to obtain permission directly from the copyright holder. To view a copy of this licence, visit http://creativecommons.org/licenses/by/4.0/.
Hussain, D., Khan, S.A., Khan, T.A. et al. Efficient liquid phase confiscation of nile blue using a novel hybrid nanocomposite synthesized from guar gum-polyacrylamide and erbium oxide. Sci Rep 12, 14656 (2022). https://doi.org/10.1038/s41598-022-18591-0
DOI: https://doi.org/10.1038/s41598-022-18591-0
Anyone you share the following link with will be able to read this content:
Sorry, a shareable link is not currently available for this article.
Provided by the Springer Nature SharedIt content-sharing initiative
Environmental Science and Pollution Research (2023)
Journal of Polymers and the Environment (2023)
By submitting a comment you agree to abide by our Terms and Community Guidelines. If you find something abusive or that does not comply with our terms or guidelines please flag it as inappropriate.
Scientific Reports (Sci Rep) ISSN 2045-2322 (online)
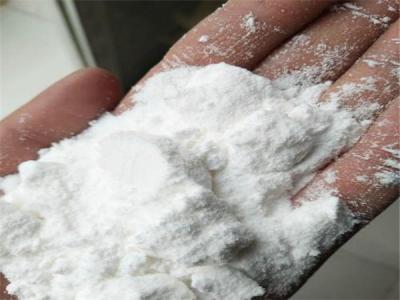
CAS 1313-97-9 Sign up for the Nature Briefing newsletter — what matters in science, free to your inbox daily.